The system will for the first time provide the capability of highly accurate remote sensing of temperature and sound velocity profiles in the ocean. There presently does not exist a remote sensing technique that can achieve the required accuracy of at least 0.1 K. This new approach will be very sensitive and large areas can be surveyed in short periods of time – a requirement vital to large scale measurement campaigns required for long term climate surveys and weather forecasting such as hurricane movements.
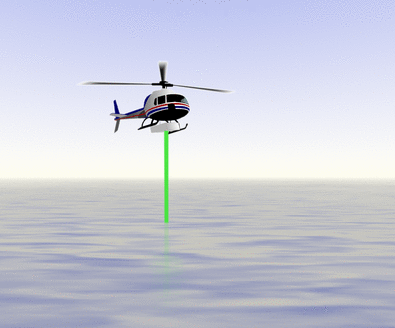
Principle – transmitter
Techniques developed by Dr. Fry (ramp and fire technique) make it possible to build powerful Nd:YAG lasers which produce Fourier transform limited pulses even in acoustically and mechanically noisy environments – a requirement essential to practical applications.
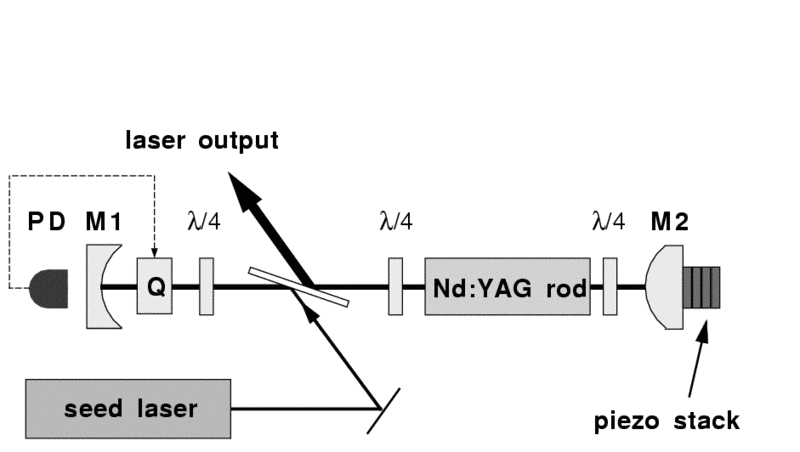
Principle – receiver
The challenge is to devise a detection scheme which fulfills three simultaneous requirements: it must be (1) capable of resolving the small Brillouin frequency shifts, (2) fast in order to provide the time resolution necessary to measure the depth profiles of the temperature and (3) rugged as it should be used in acoustically noisy environments.
Ideally suited are edge filters based on the absorption lines of molecules such as two isotopes of the iodine molecule or FADOF filters
Latest results
Transmitter System
A five-stage, frequency doubled Yb-doped fiber amplifier has been set up. For 10 ns pulses we can generate more than 0.5 mJ/pulse energies in the green spectral range.
Receiver System
Our approach for an edge filter has been successful. By the use of a modified Halbach cylinder we were able to generate an ESFADOF with the edges at the correct spacing and peak transmission up to 80%.